Author: Professor Hon Ki Tsang, FIEEE, Dean, Faculty of Engineering, WeiLun Professor of Electronic Engineering
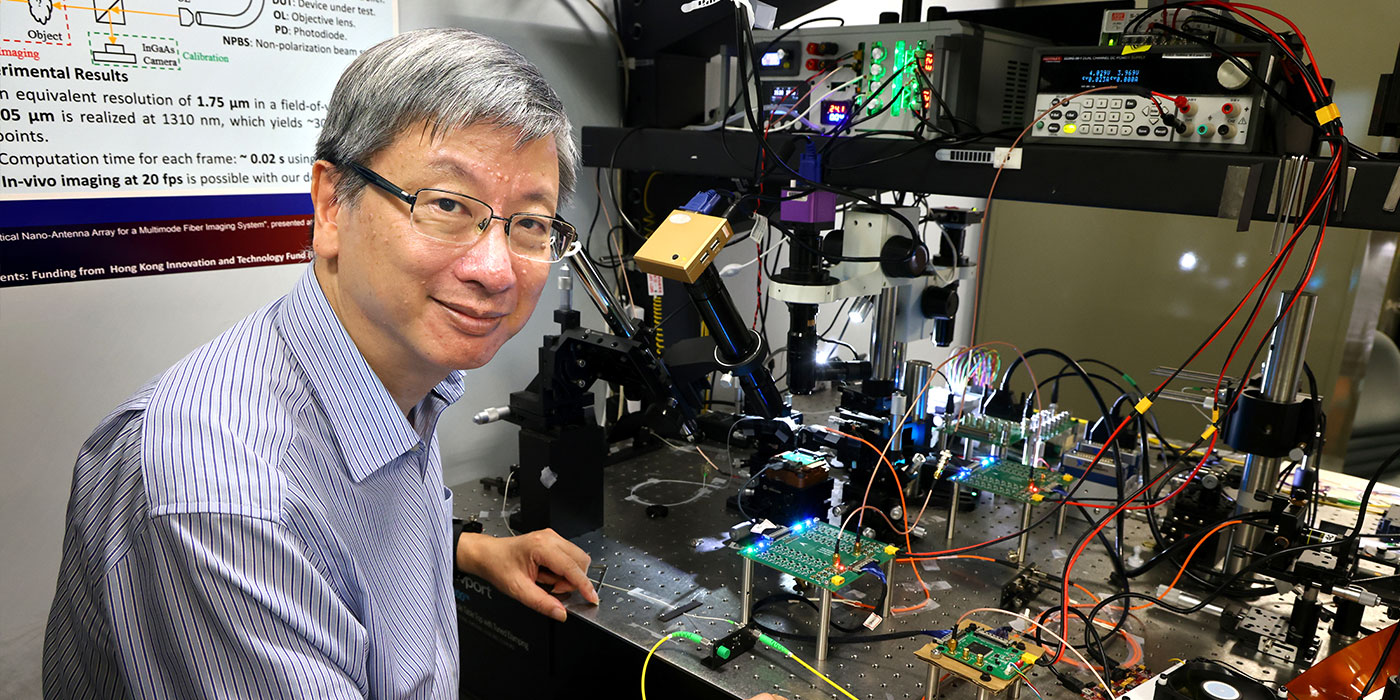
The invention of the transistor by Bardeen, Brattain and Shockley at Bell Laboratories in 1947 and the subsequent invention in 1958 of the integrated circuit by Jack Kilby and Robert Noyce changed the world. The 20th century saw rapid developments in microelectronic systems leading to the widespread use of computers, mobile electronic devices and microelectronic systems in the design and implementation of advanced technologies in virtually every sector of the economy. Recognizing the significant impact of these works, the inventors received the accolade of the Nobel Prize in Physics in 1956 and 2000 respectively. The latest generation of integrated circuits today, such as the microprocessors used in the latest mobile devices, are the apex building upon more than 30 generations of technological advances that enabled the high-yield manufacture of over 30 billion transistors within an area of a few square centimeters of single crystalline silicon. Silicon is one of the most abundant elements in the earth’s crust and has benefitted from the enormous investments over the past 70 years in research and development that has created an unrivalled knowledge base the processing of silicon for the manufacture of microelectronic chips.
The billions of transistors within a silicon microelectronics chip are connected together by copper interconnects (photolithographically patterned fine lines of copper on the silicon chip), and each copper interconnect will dissipate a small amount of energy every time the voltage of the interconnect is switched. With billions of wires in the chip, the energy dissipation adds up to heat-up the whole chip, causing large increases in the chip temperature when the maximum possible switching frequency is exceeded. In 2004 researchers at Intel estimated that the heat generated per unit volume in a microprocessor could exceed that at the core of a nuclear reactor if all the wires were switched at the maximum operating frequency of the transistors, and the whole chip would melt! The individual silicon transistor can be switched can be as fast as five hundred billion times per second (500GHz), but the problem of heat dissipation in the copper interconnects within highly integrated computer processors has limited the switching frequency (the internal clock frequency of the microprocessor) of computer processors to only about 5 billion times per second (5GHz), more than 100 times slower than the potential maximum processing speed of the individual transistors. Computer clock frequencies have not significantly improved in the last 20 years because of this problem. Silicon photonics is a possible solution to this problem. Silicon photonics is based on the use of the same fabrication technology as has been perfected in the manufacture of previous generations of microelectronic integrated circuits, but instead of using copper wires and electrical signals for carrying data, silicon photonic integrated circuits use optical “wires” which guide light waves in tiny channels of silicon known as silicon optical waveguides. Fundamentally high-speed data transmission using light does not generate heat and hence optical interconnects can potentially make computers 100 times faster.
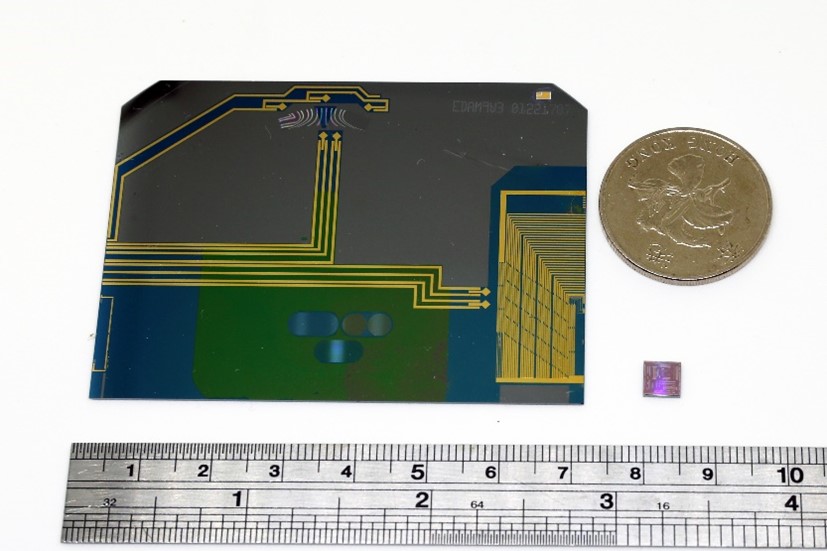
Fig. 1 (a) Comparison of the cm scale silicon photonic chip which implemented an arrayed waveguide grating optical spectrometer with 40 wavelength channels made by Bookham Technology in 2002 (left) with the latest generation silicon photonics chip designed by Prof Tsang’s team manufactured in 2023 (lower right). The 1 dollar coin is for size comparison. Advances in silicon photonics in the last 20 years has led to about one million times reduction in chip area for the same spectrometer functionality.
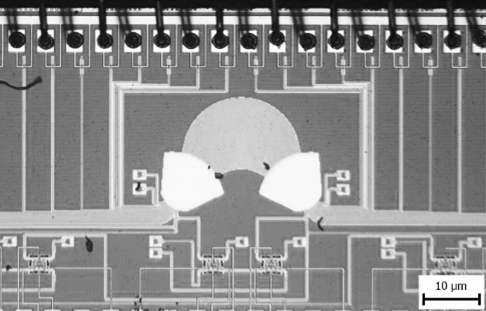
Fig. 1 (b) Close-up of part of the 2023 CUHK Silicon photonics chip capable of measuring 560 wavelength channels (note the scale bar at bottom right corner indicates a distance of 10mm is equal to 0.01mm)
The silicon optical waveguides are based on the same physical principles as optical fibers, with the light confined at the waveguide walls by the phenomenon of total internal reflection made possible by silicon having a higher refractive index than its surrounding material (typically silicon oxide or air). Silicon has a refractive index of about 3.5, which is much higher than that of glass (1.5) and hence the silicon optical waveguides are about 20 times smaller than the diameter of the core of the glass optical fibers used in optical fiber communications. My research team at CUHK has been working in the field of silicon photonics for about 25 years: Two decades ago silicon optical waveguides were limited to relatively large sized shallow etched rib waveguides which typically had waveguide widths of several micrometers , and minimum bend radii of a few millimeters resulting in quite large cm scale chips (Fig. 1) for use in optical fiber telecommunication systems for separating the different wavelengths of light in wavelength division multiplexed optical fiber communications. But with the development of higher resolution photolithography it is now possible to manufacture much smaller high index contrast waveguides with minimum bend radii as small as 3mm using 500nm width waveguides (easily with the capability of the deep ultraviolet photolithography systems widely used in silicon microelectronics more than a decade ago), and the development of the foundry business model for silicon photonics, the area occupied by silicon photonic integrated circuits have be reduced by about a million times (Fig.1), enabling more functional elements to be integrated in the silicon photonics chips to address different applications.
At the Chinese University of Hong Kong my team (Fig. 2) of PhD students and postdoctoral fellows are exploring both the immediate practical applications of silicon photonics as well as more advanced and longer term potential applications. We have developed silicon optical modulators, which convert high speed electrical signals to optical signals, and transmit data at over 100Gbaud (100 billion symbols per second), and we have demonstrated over 400Gb/s data transmission using a single wavelength of light. We have developed optical signal processors which perform high speed unitary matrix operations. These devices will be a key enabling technology for future data center optical interconnects supporting the 3.2Tb/s data transmission soon to be needed with the continued growth of the internet and the rise in demand for artificial intelligence and training of large language models.
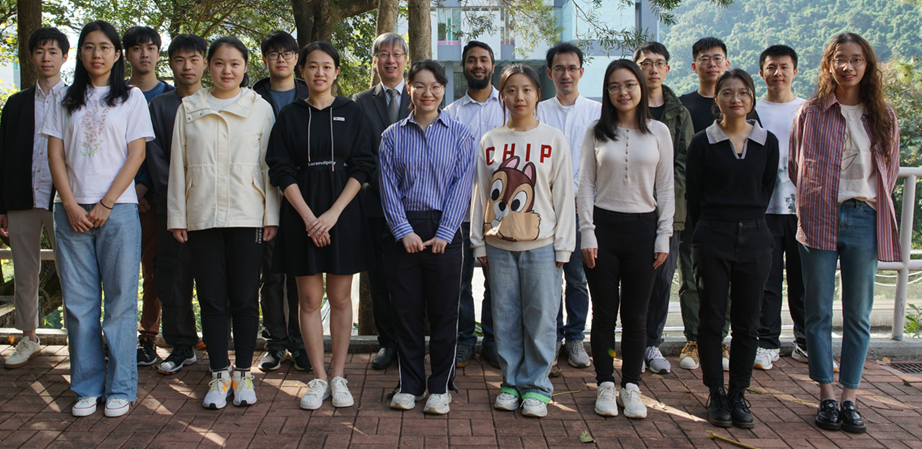
Fig. 2 Prof. Hon Ki Tsang (8th from the left) and his research team.
The enormous growth of data traffic in data centers can be met by the use of many parallel data lanes in silicon photonic integrated circuits. The use of light in photonic integrated circuits offer not only the advantage of transmitting data over much longer distances without the speed limitation from heat generation but adds extra dimensions, not available in electrical data transmission, in the transport and processing of information. For example, the optical interference of coherent light waves is equivalent to the mathematical operation of unitary matrix multiplication, and it is therefore possible to build coherent optical signal processors which can perform high speed matrix multiplications and mathematical operations such as convolution, with much lower power dissipation and latency than high-speed electronic signal processors.
Photons can have different wavelengths and polarization, which may also be used to multiplex independent data lanes in a single optical fiber in data center communications. Light is also the natural medium for sensing and silicon photonic integrated circuits can be used to process the optical signals directly for 3D imaging in optical coherence tomography and lidar range finding. And photons are inherently quantum in nature, and we can use silicon photonics to generate entangled photon pairs that exhibit non-local quantum interference, and which may find applications in quantum enhanced sensing and quantum computing, and we have already built integrated quantum interferometers operating with high quantum interference visibilities.
Leveraging on the exciting possibilities of the technology of silicon photonic integrated circuits we proposed and received approval of funding from the new RAISe+ funding scheme for our project to harvest some of the lowest lying fruits of our research in silicon photonics, specifically aimed at developing silicon photonics for advanced high-capacity data communications and silicon photonics based 3D imaging using optical coherence tomography. We have learnt from history, taking note of the success of the fabless design house model in the microelectronics industry (silicon photonics today is at a similar stage of development as microelectronics in the 1980s) and as exemplified by Qualcomm which was a startup back in 1985, we think 2024 is the right time to propose a start-up with the exact same fabless design house business model for silicon photonics. We aim to transfer our leading-edge designs in silicon photonics to create new solutions for next generation data center transceivers and optical coherence tomography imaging systems that offer significantly improved performance over the currently available systems. Silicon photonics already has a well-established market for data center transceivers. But beyond this USD$25billion market, silicon photonics may eventually be used in mobile healthcare devices that can be used for early detection of skin cancer, low cost lidar systems for use by consumers in autonomous cars, and in the longer term silicon may be used to improve the speed of high performance computers by over two orders of magnitude, and perhaps make practical quantum computing and quantum metrology devices which vastly surpass the performance of microelectronic systems. Our vision is to establish silicon photonics as a mainstream technology that becomes as ubiquitous in society in the 21st century as microelectronics and electronic integrated circuits became in the 20th century. My team’s mission is to remain at the forefront of silicon photonics and create silicon photonics system-on-chips products and modules which will empower the development of advanced, high performance and compact systems, improving performance beyond conventional microelectronics or bulk optical systems.