Prof. Emma Pickwell-Macpherson, Department of Electronic Engineering
A research team under the supervisor of Prof. Emma Pickwell-Macpherson have reached a crucial milestone towards commercializing single-pixel terahertz imaging technology for use in biomedical and industrial applications. Their single-pixel terahertz camera reached 100 times faster acquisition than the previous state-of-the-art without adding any significant costs to the entire system or sacrificing the sub-picosecond temporal resolution needed for the most sought-after applications. This valuable work was published in the world-class journal of Nature Communications, a sister journal of Nature.
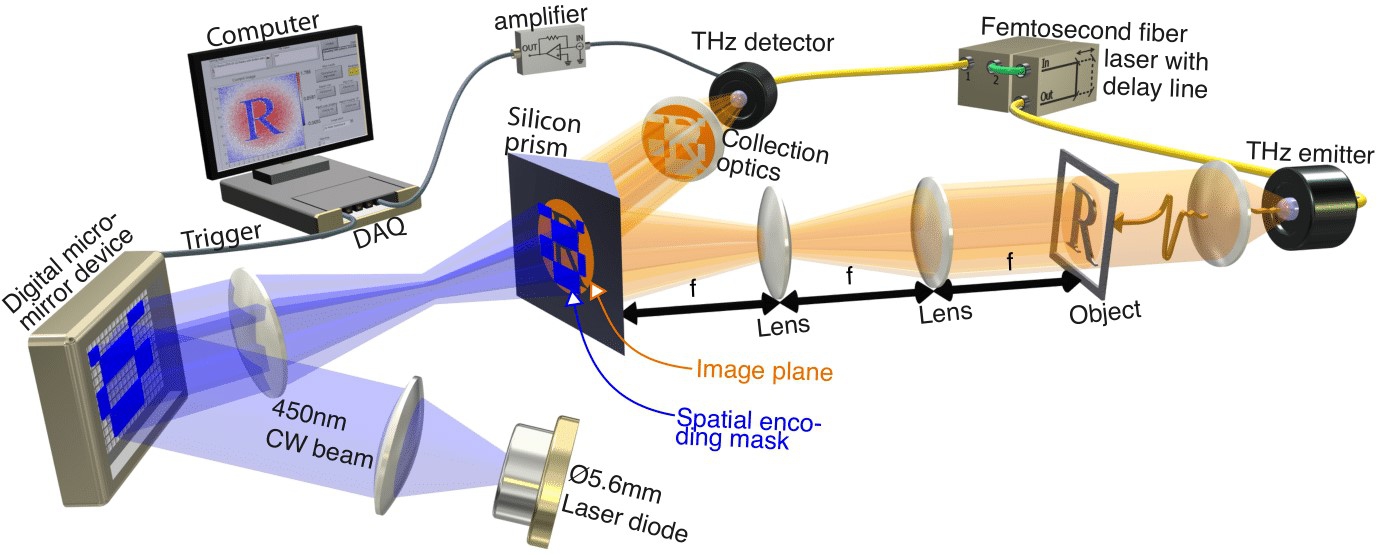
The potentials and problems of Terahertz radiation Terahertz (THz) radiation, or T-rays, sits in-between infrared and WiFi on the electromagnetic spectrum. Thus T-rays have different properties from other electromagnetic waves, most notably they can see through many common materials such as plastics, ceramics and clothes, making terahertz radiation potentially useful in non-invasive inspections. Another quality is that the low-energy photons of T-rays are non-ionizing, making them very safe in biological settings. Further, they are highly sensitive to water thus can observe minute changes to the hydration state of biological matter. This means that diseases that perturb the water content of biological matter, such as skin cancer, can potentially be in vivo detected without any histological markers.
Whilst T-rays have great potential in a wide range of applications, there is almost no commercial usage of these waves. In laboratory settings, efficient detection and generation of T-rays has occurred for last 25 years. Even still, terahertz technology is still not widely used in commercial settings as the cost, robustness and/or ease of use is still lagging behind for commercial adoption in industrial settings. For biomedical applications, clinical trials have not been performed most notably due to the equipment not being user-friendly and imaging being too slow due to the need for measuring multiple terahertz frequencies (for accurate diagnosis). Finally, equipment and running costs need to be within hospital budgets. As a result, a lot of research into terahertz technology is currently focused on developing the equipment such that one does not have to sacrifice imaging speed, reduce diagnosis accuracy or incur large costs. As a result, we have to explore alternative imaging techniques to those currently used in modern day smart phones.
The advantages of single-pixel cameras We use what is called “a single-pixel camera” to obtain our images. In short, we spatially modulate our terahertz beam and shine this light onto an object. Then using a single-element detector we record the light that is transmitted (or reflected) through the object we want to image. We keep doing this for many different spatial patterns until we can mathematically reconstruct an image of our object. We have to keep changing the shape of our terahertz beam many times which means this method is usually slower compared to multi-pixel detector arrays. However, multi-pixel arrays for the terahertz regime usually lack sub-picosecond temporal resolution, require cryogenic temperature to operate or incur large equipment costs (>350,000 USD), whereas our setup based on a single-element detector is reasonably priced (~20,000 USD), robust, has sub-picosecond temporal resolution (needed for accurate diagnosis) and operates at room temperature.
Our latest work, manages to improve upon the acquisition rate of single-pixel terahertz cameras by a factor of 100 from previous state-of-the-art, acquiring a 32x32 video at 6 frames-per-second. We do this by first considering what is the most optimal modulation geometry, second by modelizing the temporal response of our imaging system for improvement in signal-to-noise, and third by deliberating how to reduce the total number of measurements with compressed sensing techniques that do need long calculation times. In fact, part of our work shows that we can reach a five times faster acquisition rate if we had sufficient signal-to-noise ratio.
Future work will focus on improving the signal-to-noise and optimizing the software needed for accurate medical diagnosis.
|